Posted by star
on 2019-10-08 00:51:33
Hits:437
Researchers at Harvard medical school have identified a protein called epigenetic regulator that is mutated in many autoimmune diseases: SP140, which is also essential for macrophage function and intestinal homeostasis. "Many immune-mediated diseases are driven by a combination of genetic predisposition and environmental influences, and epigenetics is a key link between the two," says Kate Jeffrey, a researcher in innate epigenetic regulation at Harvard medical school.
The researchers used chip-SEQ to isolate the epigenetic reader and bind the DNA on it, and found that SP140 occupied the transcription initiation site in human macrophages. If you remove SP140 from macrophages (from mice) in the cell culture, the ability of macrophages to be activated is severely impaired. In addition, when the team knocked out the SP140 gene in the model mice's immune system, they found that the absence of this epigenetic regulator weakened the rodents' gut defense barrier, altered the balance of their gut microbes and increased intestinal inflammation.
"SP140 can change the body's response to pathogens and can make colitis worse," Jeffrey said. But we don't know what happens when SP140 becomes infected in the body.
"Initially, when we found that this epigenetic mediated factor was immunologically limited, we thought it might be an important therapeutic target because there would be no missed targets," Jeffrey said. But it turns out that if you don't have it, it causes inflammation in the gut, so you can't inhibit it."
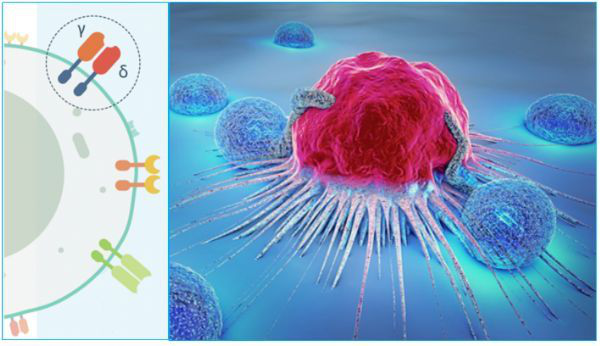
"This is a very comprehensive study that describes a previously unknown molecular mechanism of the innate immune system," said Xinnan Wang, professor of immunology at Stanford University School of Medicine.
Posted by star
on 2019-09-29 19:28:30
Hits:385
The Chinese and American research team led by the Roswell Park Cancer Institute s has linked the development of castration-resistant prostate cancer to the lack of androgen receptor expression in prostate cancer cells, identifying new therapeutic targets.
Prostate cancer is one of the most common cancers in men and the second leading cause of cancer death in men, as patients with advanced prostate cancer still develop or relapse after treatment.
For men with advanced cancer or who are unable to surgically remove the tumor, the standard treatment regimen is to use drugs to target and block the androgen receptor (AR), a protein that binds to androgen. AR targeted therapy prevents or inhibits the growth of cancer cells, but somehow their effects are always short-lived. After one or two years of treatment, many patients develop castration-resistant prostate cancer, a form of invasive and drug-resistant disease.
To unravel the mechanisms of prostate cancer resistance and development, the researchers examined AR expression patterns in 89 castration-resistant prostate cancer patients and found three different types: AR in the nucleus (nuc-AR), AR It is in the nucleus and cytoplasm (nuc/cyto-AR), and AR has low or no expression (AR−/lo).
Further studies later confirmed that cancer cells lacking AR did not respond to the treatment of the prostate cancer drug enzalutamide (trade name Xtandi). These cells are more likely to grow, regenerate, and proliferate than other AR-containing cells. Through deep RNA-Seq analysis, the researchers found that BCL-2 is a key regulator and is also expected to be a therapeutic target for castration-resistant prostate cancer.
"In order to survive under chemical castration and antiandrogen therapy, prostate cancer cells overexpress, redistribute or lose androgen receptors," the researchers said. "Our research provides a new proof of treatment strategy that not only treats advanced and metastatic prostate cancer,......
Posted by star
on 2019-09-28 19:27:03
Hits:447
Researchers at Princeton University found that a compound called Tinagl1 blocks both the epidermal growth factor receptor (EGFR) and integrin pathways and slows the spread of triple-negative breast cancer.
The researchers found that Tinagl1 inhibits two major pathways that are helpful for the aggressiveness and drug resistance of triple-negative breast cancer. Tinagl1 can inhibit both pathways in different ways, overcoming the compensation mechanism for cancer evasion treatment.
On the one hand, Tingal1 blocks the activity of a tumor-promoting protein. Mutations in this protein-encoding gene cause the epidermal growth factor receptor gene (EGFR) to soar, send growth-promoting signals to cells, and promote tumor growth and spread. However, the treatment of targeted EGFR has limited clinical efficacy, probably because cancer cells have found new growth pathways.
On the other hand, Tingal1 can also act on integrins, which are involved in regulating cell migration. Cancer cells migrate to new regions through integrins, adhere to other cells, and transform into tumors. Tinagl1 is able to target integrin signaling by interfering with focal adhesion kinase (FAK), which promotes cell migration, growth and survival.
The researchers said that the two pathways are molecularly related and can compensate each other, contributing to the invasiveness and drug resistance of triple-negative breast cancer.
The research team observed more than 800 breast tumor samples collected from human patients. They found that tumor samples with low expression levels of Tinagl1 were more advanced in cancer and shorter in survival, while patients with higher Tinagl1 gene levels were better treated. This difference was most prominent in triple negative subgroup in breast cancer.
To test whether the Tinagl1 gene prevents tumor growth and spread, the researchers engineered human and mouse tumor cells to express high levels of the Tinagl1 gene. As a result, it was found......
Posted by star
on 2019-09-24 19:13:49
Hits:438
A new study at Tel Aviv University found that an elevated level of protein called ubiquilin-4 is a new biomarker of genomic instability. The study found that ubiquilin 4 is involved in protecting the genome from DNA damage, while excessive ubiquilin 4 is harmful. When the number of ubiquilin 4 in tumor cells increases, these cells are more susceptible to genomic instability, accelerating tumor development and making them resistant to commonly used cancer treatments.
UBQLN4 deficiency leads to increased sensitivity to genotoxic stress and delayed DNA double-strand break (DSB) repair. The proteasomal shuttle factor UBQLN4 is phosphorylated
by ATM and interacts with ubiquitylated MRE11 to mediate early steps of homologous recombination-mediated DSB repair (HRR). Loss of UBQLN4 leads to chromatin retention of MRE11, promoting non-physiological HRR activity in vitro and in vivo.
Conversely, UBQLN4 overexpression represses HRR and favors non-homologous end joining. Moreover, the researchers find UBQLN4 overexpressed in aggressive tumors. In line with an HRR defect in these tumors, UBQLN4 overexpression is associated with PARP1 inhibitor sensitivity. UBQLN4 therefore curtails HRR activity through removal of MRE11 from damaged chromatin and thus offers a therapeutic window for PARP1 inhibitor treatment in UBQLN4-overexpressing tumors.
"This new biomarker provides new key information about tumor staging and grading and how patients respond to treatment," the researchers said. "Tumors with high ubiquilin 4 levels may be more resistant to radiation and chemotherapy than normal levels of tumors. But the good news is that they may respond better to other types of cancer treatment. Obviously, this is the clinician and Important information the patient needs. We hope that this discovery will provide a new tool for tumor classification, prognosis, and treatment design, emphasizing the broader impact of genomic stability on our health."
EIAAB ......
Posted by star
on 2019-09-24 00:48:50
Hits:415
Researchers at the UT Southwestern Medical Center in the United States have discovered that a new role for Perilipin 5, which is usually located on the surface of lipid droplets in cells, acts as a regulator of fat metabolism in the nucleus.
In obese people and rodent, excess fat accumulates in tissues that are not specifically used to store fat, such as skeletal muscle, heart, and liver. Researchers say this accumulation can lead to dysfunction in these tissues.
Decomposing large amounts of fat can overload the body's metabolic system, flooding small cell mitochondria, they turn fat into fuel for work or heat. The researchers said: "If you use fuel to make the plant too loaded, then it will not work well. Then, these cells only have a portion of the processed fat, which may be toxic to the mitochondria and cause type 2 diabetes. Insulin resistance that occurs in the body."
Endurance athletes - like obese insulin resistance patients, they accumulate the same amount of fat in their muscle cells abnormally. Some studies have found that they have higher levels of Perilipin 5, and this new discovery by the UTSW research team may explain Why such athletes can use the increase in fat storage to increase the motivation of exercise while avoiding the toxicity of increased muscle fat.
In cell culture and mouse experiments, the UTSW team found that when cells are stimulated to release fat stored in fat droplets, Perilipin 5 can leave the surface of the droplets and move to the nucleus of the cell where it is another protein, PGC-1α, works together to promote more and more efficient mitochondrial production. In this way, Perilipin 5 helps to match the mitochondria's ability to burn fat with intracellular fat load.
These findings may eventually lead to new therapies for obesity-related diabetes, perhaps a new drug that mimics one aspect of Perilipin 5 or its function.
EIAAB SCIENCE INC, WUHAN has developed