Posted by star
on 2019-08-21 18:45:51
Hits:454
Good cardiovascular health may reduce the risk of dementia later in life, according to new research published in the American journal of medicine. Dementia is a progressive disease that can develop 10 to 15 years before the onset of symptoms, so it is important to identify the factors that can cause its onset.
The American heart association has issued its first guidelines on cardiovascular disease. The guidelines are designed to improve diet and lifestyle through seven indicators, which could also be a potential tool for preventing dementia. To prove the point, a team of researchers at the national institutes of health and medicine collected cardiovascular data from 8,000 50-year-old men and women in the United States to examine the impact of their social behaviors and life factors on their health. Participants had no cardiovascular disease or dementia at age 50, but 387 cases were diagnosed with dementia during the following 25 years of follow-up. After taking into account other factors, the researchers found that adults who adhered to the health guidelines had a significantly reduced risk of dementia later in life. However, this study has limitations because it is an observational study and may have inaccurate self-reported measurements that ultimately lead to dementia patients missing out.
The researchers said: 'our results suggest that the risk of dementia after age 50 depends largely on cardiovascular health. To promote cognitive health, reducing cardiovascular risk should be an important strategic defense goal.

Posted by star
on 2019-08-20 19:06:11
Hits:448
Colorectal cancer is the third leading cause of cancer-related deaths in humans. The American Cancer association reported the five-year survival rate for rectal cancer patients is only 12%.
Researchers from the University of South Carolina found a potential new target for immunotherapy of colorectal cancer.
Cancer uses tolerance mechanisms to evade our immune system. T cells are immune cells that target killing cancer cells. However, they are also classified into different types, such as a regulatory T (Treg) cell, which ensures that we maintain tolerance to our own cells. Cancer cells increase the presence of Treg cells and are prevented from being killed by other types of T cells.
In a mouse model of colitis, deletion of the GARP gene on Treg cells prevents the intestinal immune system from maintaining optimal tolerance. Without GARP, Treg cells do not effectively suppress the immune system, and they also enter the intestine less. In addition, the team also found that in the mouse model of colon cancer, the deletion of GARP on Treg cells reduced the tumor in mice by half.
"In preclinical cancer models, mice lacking GARP on Treg cells performed better and more T cells infiltrated the tumor," the researchers said. "This seems to happen only in the intestines. When other parts (such as the skin) induce cancer, there is no difference in the presence or absence of GARP on mouse Treg cells."
Studies have shown that another major factor involved in mediating Treg cells is TGF-β. The cells release TGF-β, GARP is involved in TGF-β activation, and TGF-β and GARP together regulate the migration of Treg into the intestine.
If researchers can figure out how colon cancer cells increase the homing of intestinal Treg cells, they can block this homing signal, allowing the immune system to detect cancer and eradicate cancer.
Currently, they believe that the cell surface protein CD103 may be a homing signal. "When the surface......
Posted by star
on 2019-08-19 18:53:24
Hits:435
In recent years, interest in the Warburg effect (abnormal metabolism of cancer cells) has re-emerged. The rationale for this treatment strategy is to starve tumor cells while allowing healthy cells to survive alone.
Researchers at the University of Emery's Winship Cancer Institute have identified a transport enzyme called ASCT2 as a target for anticancer drugs, which is responsible for bringing amino acids into cells. Deletion of the gene encoding this enzyme prolongs the survival of invasive leukemia (AML/acute myeloid leukemia) mice - from 45 days to more than 300 days.
ASCT2 can transport some amino acids, such as glutamate. In leukemia cells, the absence of ASCT2 has an overall effect on cellular metabolism, interferes with leucine influx and mTOR signaling, and induces apoptosis.
The study found that in mice, the gene encoding ASCT2 can be deleted without substantial disruption of blood cell development. However, under the pressure of chemotherapy or radiotherapy, the ability of this mouse to restore white blood cell count is indeed slower.
"So far, research on finding therapeutic targets in amino acid metabolic pathways has been slow. These targets must kill cancer cells, but retain normal cells. ASCT2 is dispensable for normal blood cell development, but it is important for the development and processes of leukemia." the researchers said. “Although research strongly recommends ASCT2 as a target for leukemia treatment, clinical trial researchers need to carefully combine ASCT2 inhibitors with chemotherapy.”
EIAAB SCIENCE INC, WUHAN has developed ASCT2 protein, antibody and ELISA kit.
Welcome scientific research workers to choose and purchase.
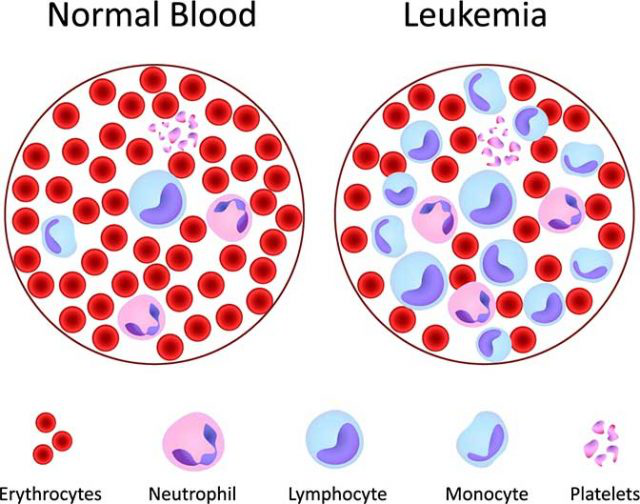
Posted by star
on 2019-08-18 19:08:33
Hits:437
A team led by Dr. Manuel Litchman of the University of California School of medicine found the transformation of melanoma cells in samples of melanoma patients. The mechanism for this transformation is that growth-restricted cancer cells in the epidermis transform to attack other vital organs.
The tumor grows in the upper surface cortex, usually in a superficial proliferative phase. But when they grow and touch the deep layers where fat is present, the invasion of the tumor changes from horizontal to vertical. Manuel Litchman and his team co-cultured melanoma cells with adipocytes and identified adipocytes as involved in the transformation of melanoma cells. The researchers found that fat cells transfer cytokines that affect gene expression to melanoma cells. When these cytokines bind to receptors on melanoma cells, they signal to suppress the expression of a receptor called TGF- beta. TGF- beta receptor concentration in fat cells decreased, so that part of the TGF- beta signal cannot bind to the receptor, tumor absorption of TGF- beta signal, further stimulate melanoma cells, making it more invasive.
At the same time, the researchers demonstrated that the transformation process is reversible. When the fat cells in the melanoma cells are removed, the activity of the cancer cells decreases, or even quiets down.
Based on the new findings, the researchers experimented with therapies that inhibit cytokines and TGF- beta, which inhibit metastasis and restore melanoma cells to their "resting state."
Dr. Litchman concludes, "This finding should be used as a foundation for new drug development and to prevent the metastasis and spread of melanoma."
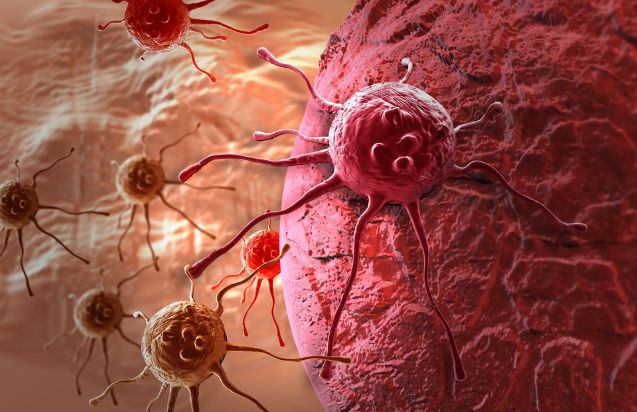
Posted by star
on 2019-08-15 18:28:10
Hits:446
Microtubules play an important role in cell secretion, transporting intracellular substances to the cell membrane for secretion. Professor Clea Barcena of the University of California, Berkeley, and her team found that microtubules act as cell "monitors" to accurately control insulin secretion in pancreatic beta cells and found that this monitoring disorder may lead to beta cell dysfunction, which in turn leads to type 2 diabetes. Therefore, the research team took the regulation of microtubules targeting insulin secretion as a new research direction. This research direction may provide a new way to treat diabetes.
Professor Clea Barcena and her team used a model of pancreatic beta cells to study the function of microtubules and explore how they "transport" substances such as insulin particles from inside cells to outside. The researchers first used the compound to destroy the microtubules, then stimulated islet beta cells with glucose and measured insulin production. Because microtubules are destroyed, insulin production is theoretically reduced. But they measured a large increase in insulin. "In any model we tested, the destruction of microtubules increased insulin production," Barcena said. "Do microtubules inhibit secretion?"
To explain this, the researchers used ultra-resolution microscopy to find that in beta cells, microtubules are not a straight transport channel, but a complex network.
"Insulin particles travel across a network of microtubules, a behavior that regulates the number of particles to prevent oversecretion," Barcena said. Based on the findings, the researchers suggest that disrupting microtubules, delivering targeted drugs to the pancreas and increasing insulin production, could be a way to treat diabetes.
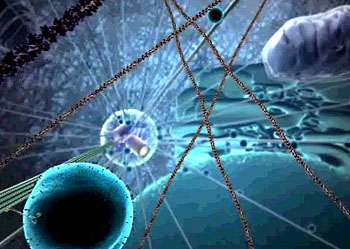